Research Synthesis on Relative Fitness of Antibiotic Resistance
by Sophie Miller
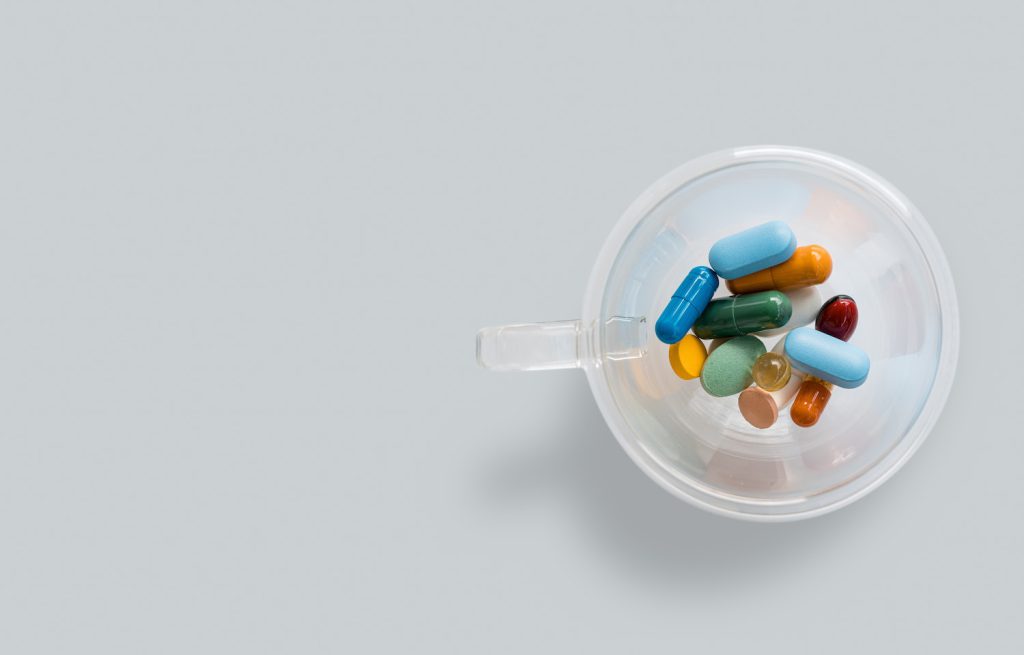
Resistant strains of bacteria are on the rise, which limits the effectiveness of antibiotic treatments. By understanding the specific mutations that create resistance and how the mutations affect the metabolic pathways of the different strains, we can better learn how to minimize resistance. This paper will also explain how the genetic background of bacterial strains affects the ability to maintain resistant mutations. I will be examining what is known about resistant mutations and what needs to be further researched to better treat bacterial infections in the future. The focus is placed on reproductive fitness and the competitive costs associated with the different mutations. Hopefully, by analyzing these factors scientists can find a way to control the ability of bacterial strains to retain the mutations providing resistance in the absence of antibiotics.
antibiotic resistance, competitive cost, relative fitness, genetic variation, genotype‑by-environment interaction
Background and Relevance
Bacterial infections are a major source of illness among humans. These infections are caused by a class of organisms known as bacteria. Bacteria are microscopic, single-celled, prokaryotic organisms that, when inhabiting another macroscopic lifeform such as humans, can cause harm to their host environment. When bacteria invade a human body, they reproduce quickly, and their presence causes damage to the nearby host cells through their sheer number or the release of toxins.
As we have gained a better understanding of the world around us, we become better equipped to solve new problems as they arise. Specifically, we are working on finding a better solution for treating bacterial infections. Today, we rid ourselves of harmful bacteria with antibiotics. These medicines are used to target different aspects of the bacteria’s metabolism or other necessary functions so that they can be killed while hopefully causing minimal damage to our own healthy cells.
The problem with this solution is that as time goes on we are finding mutant strains of bacteria that are becoming resistant to the current set of antibiotics. The mutations appear to be caused by adaptation but may be occurring more rapidly due to over-prescription of antibiotics in general. Going forward, we may need to find a new kind of treatment for bacterial infections or a more efficient management method to prevent total antibiotic resistance.
One way for scientists to understand why bacteria are becoming more antibiotic resistant is to examine the relative fitness of bacteria with different mutations. Mutations are changes in the genetic code that create different proteins altering how an individual may react to different stressors. Relative fitness refers to how individual bacteria of the same species can have different reproductive success correlated to the specific mutation variant known as an allele that they have for a given gene. Early research shows that some forms of resistant bacteria have an innate reproductive cost when compared to wild-type bacteria in an environment absent of antibiotics (Andersson 2006, Anderson 2010, Gagneux 2006, Melnyk 2015). Reproductive cost is when an allele is deleterious in the given environment. In order to prevent the antibiotics from working properly many bacterial resistance mutations will alter their cell membrane to prevent the drug from binding. This change is beneficial when antibiotics are present but could become detrimental in their absence if the change hinders the bacteria’s ability to uptake nutrients. When we compare the differences in reproductive costs for two allele variants, we refer to the difference as the competitive cost. In an environment that lacks antibiotics, the non-resistant bacteria produce more viable offspring than the resistant strains. This is the competitive cost that antibiotic resistance requires and it could hint that there is a potential for antibiotic cycling. Antibiotic cycling is when there is only a single set of antibiotics that doctors may prescribe to patients at any given time. After several years that set would be switched to a different set to prevent widespread antibiotic resistance. Removing some antibiotics from use for several years could provide the nonresistant bacteria an opportunity to outcompete resistant strains before the original set of antibiotics is reintroduced into a population.
The wider scope of bacterial resistance research is to study the overarching evolutionary mechanisms of how species adapt to better handle new and stressful changes in an environment. By using bacteria as a test species, we may better come to understand the mechanisms of evolution that occur under complex and changing environments, specifically, the continual cycling of a stressor and its removal. Using bacteria as a test species is conducive to understanding long-term evolutionary adaptations due to their rapid reproductive cycle. The short generational timespan allows for the observation of many generations within a short and observable timespan. The alternating presence or lack thereof of antibiotics constantly changes the environment, which allows evolutionary researchers to test how genetic variation is affected by ongoing environmental change. Some genes are only beneficial in specific environments yet harmful in others. By allowing for research into the competitive costs of different alleles for one species, we can better understand how traits that appear deleterious in a benign environment become common among different populations.
Previously Investigated
Gagneux (2006) studied genetic differences between multiple strains of M. tuberculosis that had different mutations within them that lead to resistance against the antibiotic rifampin. There were variations among the genetic mutations that caused this resistance in M. tuberculosis. This variation was shown to affect the relative fitness of the mutated bacteria in comparison to one another when rifampin was absent. A strain of M. tuberculosis that had a different genetic background than that of the original experiment was also analyzed so the two backgrounds could be compared. The different variations of rifampin resistance were tested against one another and against the same mutations from the secondary strain with the alternative genetic background (Gagneux, 2006). The result of this study showed evidence that there may be gene-by-environment interactions. Gene-by-environment interaction is when different genotypes, the specific alleles, respond differently to changes in the environment. It is shown by the differing effects of the same mutation in the context of various genetic backgrounds which is the context of the bacteria having the same mutation causing antibiotic resistance but that have other genetic differences between them that are unrelated to the resistance. The combination of the differences due to the genetic background along with the different mutations themselves that provide rifampin resistance create a multitude of different competitive costs for rifampin resistance within various strains of M. tuberculosis.
It is important to note that the reproductive cost of resistance appears to be more relevant to its prevalence than the rate of a resistant mutation occurring. Having higher competitive costs limit reproduction despite relatively common appearances of different mutations that provide antibiotic resistance (Andersson, 2006). The frequency of a beneficial mutation occurring is less important than the degree of impaired fitness that the mutation would give to the bacteria when no antibiotics are present. The Gagneux (2006) paper illustrates that there are multiple ways that antibiotic resistance can form in a single species of bacteria despite all of the mutations occurring within similar genes. It is established that a genotypic, otherwise known as an allelic, difference does cause differences in the competitive cost despite the same phenotypic, or observable, appearance of antibiotic resistance. Bacterial genetic background also plays an interconnected role with the genotype to influence the relative fitness of the bacteria. These factors were studied for a specific bacterial species but to be relevant to the medical field it would be best to compare these factors across multiple species and different antibacterial resistances, which is where Melnyk (2015) becomes useful.
The specific mutation and genetic background affect the competitive cost of antibiotic resistance across multiple bacterial species. The drug class of the antibiotic also appeared to influence the competitive cost as resistance to certain classes seemed to come at a much higher cost (Melnyk, 2015). This could imply that some classes of antibiotics target more highly refined metabolic pathways of bacteria in which any mutation that would protect the bacteria from the antibiotic is likely to severely compromise the metabolomic function of that bacteria. There is a lack of data about individual bacterial species, so that does limit the amount of information that can be inferred from this comprehensive data set. However, several of the mutations had very high relative fitness in comparison to others, leading to a widespread range of fitness within the limited data set presented (Melnyk, 2015). From these findings, it can be concluded that the degree of metabolic change to confer resistance varies greatly causing an unclear linkage between the two when it comes to limiting the effect of antibiotic resistance.
Competitive cost does affect some bacteria to the point that after antibiotics cease to be used, the mutation that created the resistance does decrease in its frequency within a population (Andersson, 2010). However, this alone does not offer a way to decrease antibiotic resistance within populations as there is evidence of compensatory mutations and co-selection that may keep the resistant mutation within the population despite its initially decreased relative fitness (Anderson, 2010 and Melnyk, 2015). Compensatory mutations are secondary mutations that help to minimize the cost of resistance and sometimes can even return fitness to levels found before the resistant mutation arose. The ability to form compensatory mutations allows for the bacteria to maintain its resistance with the same fitness as susceptible bacteria, which removes the competitive selection (Andersson, 2006). Co-selection is when resistance becomes a linked trait to that of another highly favorable allele. With this linkage, the resistance mutation could stay within the population despite its somewhat deleterious effects on fitness when there are no antibiotics present (Andersson, 2006). These factors are what weaken the possibility of using antibiotic cycling as a method of decreasing antibiotic resistance. During the use of a single set of antibiotics, it is likely that the bacteria will mutate in a way that there is no longer a high cost of fitness associated with the mutations providing resistance. If any of the resistant strain is still present when it is time to reintroduce that antibiotic back into medical treatment then there will be an exponential increase in the number of resistant bacteria, which would leave us in the same if not worse situation to fight off increasingly resistant strains of bacteria. It is also important to note that thick walled bacteria also known as Gram-positive bacteria had a trend of higher overall competitive costs when compared to the thinner-walled cells of Gram-negative bacteria (Melnyk, 2015). This could be investigated to see if the wall thickness of a bacterial cell increases the need for a greater degree of metabolic mutation to convey any antibiotic resistance.
Melnyk (2015) builds on the information found in Gagneux (2006) as it confirms that a multitude of bacterial species have different competitive costs for the same mutations across different genetic backgrounds. There is also evidence that there are differing relative fitnesses based on the specific mutations within an assortment of bacterial species. The degree of competitive cost is what matters most in the reversibility of antibiotic resistance, but, even with high selectivity, if a small fraction of resistant bacteria remained, then a resurgence of the resistant strains would reemerge with the reintroduction of that antibiotic (Andersson, 2010). Due to the evidence that there are mechanisms that would maintain the presence of the resistant mutation thanks to compensatory mechanisms or linked traits it is unlikely that the resistant strains would even become all that rare within a population of bacteria, further limiting the hope for antibiotic cycling as a method of controlling resistance (Andersson, 2006).
Unanswered Questions
Gagneux (2006) mentioned that no one has yet investigated if resistance and competitive cost for one antibiotic was affecting those factors for a second antibiotic. It is important to know that many bacterial species can gain resistance to multiple antibiotics. All of Gagneux’s rifampin-resistant M. tuberculosis were also resistant to isoniazid or streptomycin and isoniazid. However, there was no information as to whether there was a difference in the average cost of resistance between the two types of co-resistors.
The effect of long‑term incubation of the bacterial infection could have influenced Gagneux’s (2006) findings in the clinical observation. With long-term incubation in vivo, the patient’s bacterial colony could have altered its mutations or had interactions among the matrix of the body’s innumerable intermingled lifestyle, environmental, and genetic confounding factors. When observing clinical patients to see if the results found in a laboratory setting were transferrable to in vivo, it was found that there was a notable difference between the in vivo and in vitro experiments (Gagneux, 2006). In vitro experiments are much more controlled than the in vivo ones, with many factors being impossible to account for in a living subject with its own unique genetic and environmental background. The in vivo experiments reported findings of higher competitive fitness than what had been documented in the in vitro experiments, showing that there is a knowledge gap that had not been accounted for in the in vitro experiments. There must be some aspect within the body that the resistant bacteria are better adapted for that was not present in a simple petri dish environment. While there are notable differences between in vitro and in vivo, the trend of the relative fitness of different mutations for resistance is consistent between the two environmental experiments (Andersson, 2006).
There is little data on the genomics of multi-resistant bacteria and how closely related the different resistant conferring mutations are. If the mutations are closely linked, eliminating antibiotic use to minimize the resistance of one will have little to no effect of eliminating its allele from the population even with a higher competitive cost due to the linking effect of the untargeted mutation (Andersson, 2006).
No one has yet assessed how different environmental factors and stress placed onto bacterial populations may affect the relative fitness of antibiotic resistant bacterial cells. Resistance may also affect bacterial survival time on different surfaces or within air particles, which could affect the rate of human infection. There is also minimal data to compare in vitro data to in vivo experiments except to say that there are substantial differences with no way to make a mathematical correlation to account for the differences present within the body (Melnyk, 2015).
Next Step in the Research
Thanks to past research, it is evident that bacteria are evolving new forms of resistance to antibiotics. This resistance comes in varying levels of competitive cost for the bacteria and therefore some mutations appear to have no cost at all. The lack of reproductive cost would lead to two branches of potential solutions: continuously create new antibiotics or find a new way to target and destroy harmful bacteria in our bodies.
New antibiotics would create a temporary solution that would constantly need to be changed over time as new mutations continue to arise and form low to no-cost resistance and become linked to extremely beneficial or highly conserved genes. Conserved genes are those genes that are highly optimized to be the most effective version possible and are often tied to vital pathways that lead to death if they are altered in any way. While this solution is temporary, it does offer time to further investigate more areas of research before immediately jumping into recreating the wheel.
A more permanent solution would be to create a drug that could target prokaryotes specifically without harming our eukaryotic cells. There are clear differences between these two types of cells, which could be leveraged against bacteria. One way of doing this could be to target the cellular differences like a specific, highly conserved bacterial protein that is essential to its reproduction, possibly its cell wall or the flagella. If the target is highly conserved enough so that there is no variation within bacterial populations, it would be highly unlikely that the bacteria could mutate in a way that does not irrevocably damage function and fitness yet is still able to confer resistance to the treatment. For a new drug to be successful, the target should only be found in prokaryotes to prevent any harm coming to our eukaryotic cells and that it is so highly conserved that there are practically no nonsynonymous changes that can be viable to prevent the possibility of mutation.
When considering where this research should be taken next, scientists should be advised to see if there are traits other than antibiotic resistance that could be evaluated for ongoing changes to the environment. If there are some heat-resistant bacteria strains, what happens when they are alternatively exposed to hot and then cold temperatures? By delving deeper into the concepts of alternating environments and how genetic variation is affected by these ongoing environmental changes then that information could be extrapolated into other species to be tested. We could look into creating stronger agricultural crops that both survive drought better and can still survive in areas of higher rainfall. There are many circumstances in our world that revolve around a constant cycle of changing environments. If we could better understand the process of mutated resistance arising and how the relative fitness can be raised or linked to other beneficial traits, then we could be better prepared for rapid changes that may happen as we continue to alter the global environment around us. With the use of systems such as CRISPR, we can create rapid evolutionary changes to organisms around us if we only know where to start. The first step of this process is to better understand how species naturally evolve resistance to environmental stressors and compensate for decreased fitness present when that stressor is absent.
Works Cited
Andersson, D. I. (2006). The biological cost of mutational antibiotic resistance: any practical conclusions? Current opinion in microbiology, 9(5), 461-465.
Andersson, D. I., & Hughes, D. (2010). Antibiotic resistance and its cost: Is it possible to reverse resistance? Nature Reviews Microbiology, 8(4), 260-271.
Gagneux, S., Long, C. D., Small, P. M., Van, T., Schoolnik, G. K., & Bohannan, B. J. (2006). The competitive cost of antibiotic resistance in Mycobacterium tuberculosis. Science, 312(5782), 1944-1946.
Melnyk, A. H., Wong, A., & Kassen, R. (2015). The fitness costs of antibiotic resistance mutations. Evolutionary applications, 8(3), 273-283.
Citation Style: APA