The Effect of Increasing Water Salinity on Magnolia virginiana
by Anvith Reddy
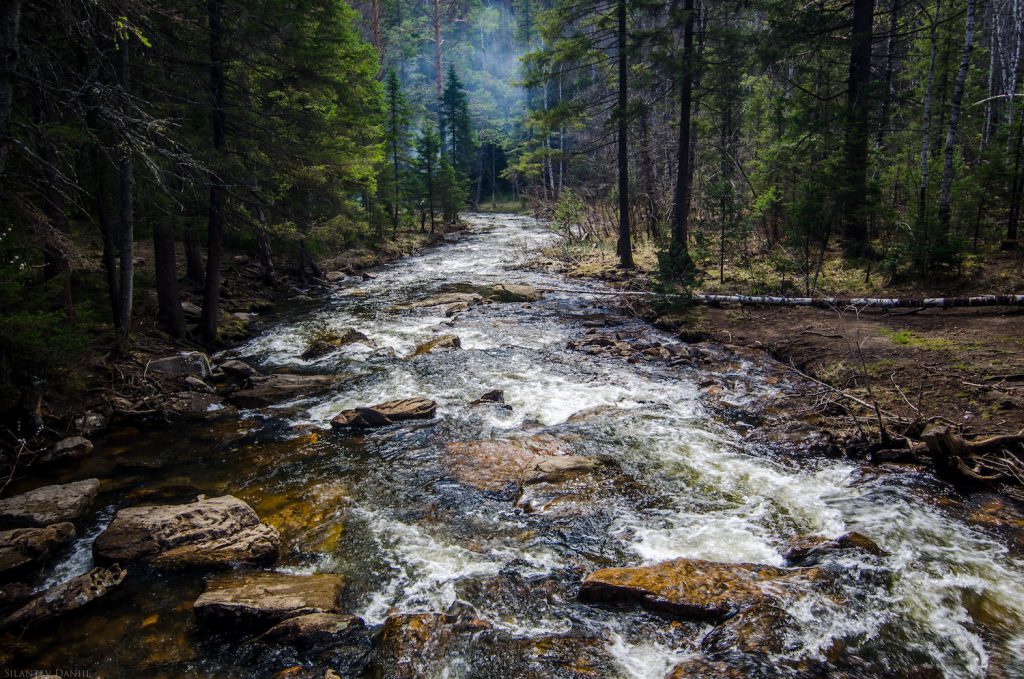
Runoff water is a major contributor to pollutants entering freshwater systems. Despite efforts to reduce runoff, recent studies have predicted a drastic increase in pollution due to runoff which introduces the danger of manmade salts entering water tables. Salinity levels have vast impacts on plant function including stomatal changes, growth regulation, and water uptake. Here, we aimed to measure the effect that increasing salinity levels would have on a representative freshwater organism, Magnolia virginiana. We tested water uptake on the principle that this is a trait essential to survival and that it would influence whether offspring could survive and transfer their genes to offspring. We predicted that the plants would uptake less water as salinity increased in order to preserve their vascular structure, and experimentally tested our Magnolia branches with a potometer and three levels of salinity: no salinity (control), five percent, and ten percent saline solutions. When graphed, the results of our study indicate that the M. virginiana branches took up a lower amount of the saline solution as the salinity increased with a negative trend in the data. Although this experiment supported our hypothesis, more research is needed to understand the complex mechanisms regulating salt uptake, along with the important impact it has on freshwater tables.
KEYWORDS: Plant Biology, Environmental Stressors, Environmental Pollution, Water, Salinity, Magnolia virginiana, freshwater ecosystems
Introduction
Salinity levels in freshwater systems are increasing at alarming rates, posing a severe risk to flora and fauna globally. In areas where chlorine infiltration has not typically been a major issue (5%), current studies by Kaushal et al. (2005) have shown a 20% increase in recent years. On top of this, during the winter there is an observed hundred-fold increase of salinity in previously pristine streams (Kaushal et al., 2005). At the current rate of salinity increase, freshwaters across the nation and world could become hazardous to plant and human life alike (Silva et al., 2010).
Saltwater intrusion is a problem primarily because it increases the concentration of chloride ions in solution. While chlorine can be beneficial in small amounts, at higher levels it can lead to plant toxicity and leaf damage over time. At salinity levels of just 30 parts per million (ppm), studies have shown damage to plants systems as salinity can adversely affect plant growth rate (Nishida, 2009). Furthermore, an alarming salinity level of 1000 ppm can have lethal effects on not only aquatic plants but also vertebrate species, such as amphibians (McKee et al., 1989). And, as human activities cause increasing salinity levels, we can expect more and more plant species to be affected over time. In order to understand these changes, and perhaps mitigate some of the effects, it is crucial to better understand the mechanism by which salinity affects the survival and reproduction functions of plants.
In this study, we explore the effects of salinity levels on water uptake functions. It is known that plants are able to sense increasing sodium levels and signal stomatal closure and leaf expansion inhibition. However, water uptake is also a critical function regulated to satisfy a variety of life needs in plants. Plant species usually prefer environments with more dissolved solute within their cells in order to maintain their vascular structure and rigid cell walls (Strotmann, 2000) as this helps them grow upward and reach sunlight. Because of this, plants likely evolved adaptations to regulate the uptake of water in order to balance their inside and outside solute levels to keep their cell structure intact. We hypothesize that one of these adaptive mechanisms is to regulate water uptake rates in different salinity levels. To test this hypothesis, we conducted an experiment to test the effects of different salinity levels on water uptake rates in M. virginiana plants. We chose the Magnolia plant (M. virginiana) for our study as it is adapted to low-lying freshwater environments, the same environments most susceptible to saltwater intrusion and because Magnolia have been shown to be sensitive to environmental stressors like changing pH and salinity levels (Thien, 1974). We predicted that water uptake in Magnolias would decrease as water salinity increased, as this would allow the plant to maintain its internal solute balance.
Water uptake is a crucial function for plant survival and understanding the effects of salinity on water uptake has broad theoretical importance. The data we obtained from magnolias may be applicable to a wider variety of freshwater plants in similar ecosystems. Given current rapid environmental changes caused by sea level rise and pollution, understanding the effects and mechanisms of salinity on water uptake could have environmental significance and practical applications as well.
Methods
To test our hypothesis that plants will down-regulate water uptake as salinity levels increase, we measured water uptake rates for Magnolia in different saline solutions. In terms of the dependent variable, M. virginiana, we selected branches of comparable size with a thickness optimal for the tubing of the testing device, approximately one centimeter. To standardize our samples, we trimmed each branch so that it had the same amount of leaves (5) and used parafilm to cover exposed sections to protect from water transpiration. It is, however, a possibility that stress from trimming the branches and leaves could have affected transpiration. To account for this created stress, we allowed plants to rest for approximately 20 to 30 minutes during preparation of the 0%, 5%, and 10% saline solutions. The Magnolia branches were allowed to come to room temperature from the hotter environment they were harvested from and adjust to the climate-controlled environment of the lab.
Our independent variable was the salinity level. We used three different salinity levels (0%, 5%, 10% w/w) and recorded changes in the dependent variable using a potometer. To get the most accurate and replicable results across our trials we eliminated confounding variables by using water and non-iodized sea salt obtained from the same source, as water or salt from different sources might have contaminants and trace chemicals that might skew the data.
To measure water uptake of leafy plant shoots, we used a potometer. First, we filled the bottom section of the potometer with a substantial amount of water. Next, we rinsed the tubing with the solution we were going to be testing. This rinsing also provided a way to get rid of any trapped air bubbles that could inhibit water uptake. We then trimmed 1 centimeter off of the Magnolia branches under water to expose fresh tissue and prevent air bubble formation. We inserted the branch into the tubing, adjusted the clamps, and started the timer immediately. We carefully watched the plant and took (1) an initial water reading, (2) a reading at the 5-minute mark, and (3) a final reading at exactly 10 minutes. After recording the data, along with the mass of the Magnolia plant used, the plants were responsibly disposed.
Results
A total of thirteen Magnolia branches were tested in our experiment over three salinity levels and two days of experimentation. We performed three trials with three different Magnolia in each condition and observed a negative trend corresponding to increasing salinity levels and decreasing water uptake. This indicates that plants down-regulated water uptake when exposed to increasing salinity.
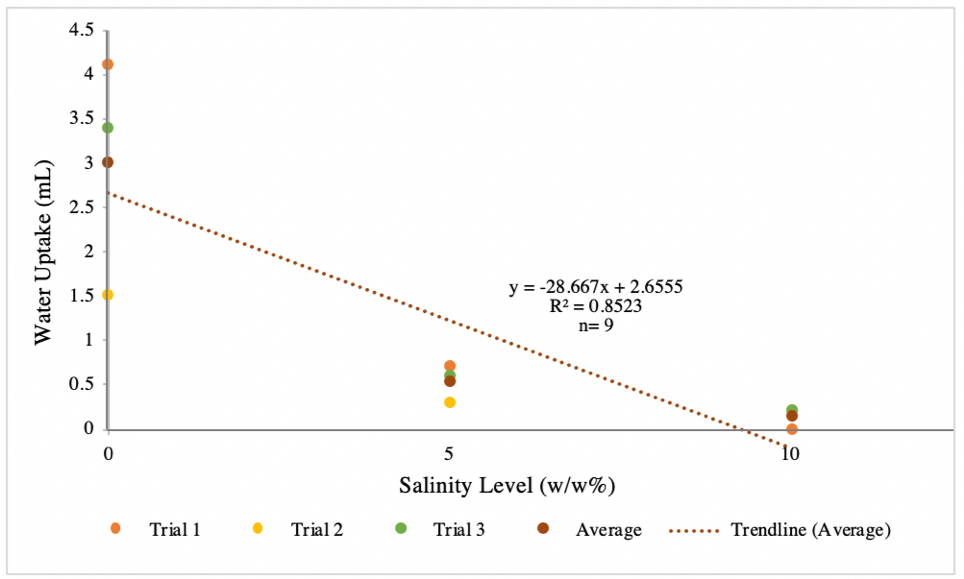
Figure 1.1 Water uptake (mL) as a function of increasing salinity level (w/w%) in Magnolia (M. virginiana) Branches. Pictured above are trials 1-3, nine plants total with each uptake level measured at 10 minutes. The dotted line is a trendline of average water uptake values. The calculated water uptake (final water level – initial water level) was the dependent variable and was graphed against our three salinity levels, the independent variable.
With the data, we calculated the Pearson’s correlation coefficient for our sample and found moderate to strong correlation between salinity and water uptake with a reported R-squared value of 0.8523. We believe our R-squared value, the correlation of our independent and dependent variables, and statistical confidence would increase with a greater number of trials at each salinity level. Our control group was the 0% w/w salinity against which we compared the rest of our data. On average the control group of Magnolia took up about 3mL of water. However, we had to exclude two trials at the 0% percent level as the Magnolia took up either 0 or only 0.1 mL of water; this was an experimental/machine error resulting from improper clamping of the potometer.
Table 1.2 Water uptake depicted in a table against the range of values for each salinity level. The range was calculated by subtracting the lowest water uptake value from the smallest uptake value. There is a general negative trend.
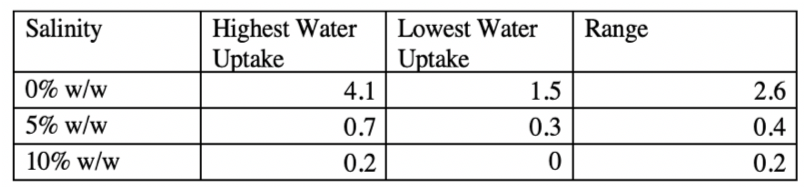
We recorded the mass of each Magnolia after trimming the leaves (Tables 1.3) to determine if there was any correlation between mass water uptake at each salinity level and if mass was a confounding variable. The average mass of Magnolia used at each salinity level was within ten percent of the average mass used at other salinity levels, indicating this difference was not large enough to influence the trend in our data.
Table 1.3 The mass of each Magnolia was recorded for each trial and weight % of salt. The mass was recorded after trimming and we carefully labeled each Magnolia for accurate testing.

Discussion
We hypothesized that the Magnolia branches had functional mechanisms to regulate water uptake in different salinity levels and maintain a solute balance across cell membranes (Negrao, 2017). We predicted that branches would uptake less water with increased salinity levels. Our results showed that as salinity increases, water uptake decreases. This is in accordance with our predictions and research by Motos et al. (2017). The observed negative correlation between water uptake and salinity level supports our deeper hypothesis that magnolia plants may have evolved mechanisms to regulate saltwater uptake and thereby protect against the harmful effects of high salinity concentrations on plant physiology. This is important because direct exposure to high salinity can lead to fatigue of cell walls in plants (McKee, 1989) and may impede the proper functioning of plant stomata (Nishida et al., 2009). However, the adaptive solution of reducing water uptake under saline conditions has several tradeoffs. For example, with less water uptake, there is less photosynthesis and, thus, less plant growth. This tradeoff has been demonstrated by previous studies showing that increasing salinity levels have a correlation with a decrease in biomass (McKee, 1989).
Although some studies have measured transpiration rates, growth rates, and gas exchange in response to salinity increase, there has not been much in terms of water uptake in Magnolia virginiana. Our research fills this gap by demonstrating the negative effects of salinity levels on water uptake, however, due to time constraints we were only able to perform up to three trials for each salinity level. This small sample size greatly limits our confidence in the accuracy of our results. In addition, we only tested two salinity levels (5%, 10%) besides the control group. Future testing could use a wider spectrum of salinity levels to get a clearer picture of the trend between salinity levels and water uptake. Our research is limited because it examines only one species while other freshwater plants might be affected slightly differently.
This research may prove significant for predicting long-lasting changes to low-dwelling freshwater plants and ecosystems. Our study indicates that salinity may adversely affect water uptake in plants which could contribute to a loss in plant biomass. As primary producers, plants have a critical role in the ecosystem. Salt water intrusion can be attributed to many sources, including contaminants in freshwater runoff produced by humans as well as global climate changes such as rising sea levels. These pollutants come from a variety of processes such as roadway de-icing salts and other factory byproducts that result from modern production methods as well as natural sources such as rising sea levels. Reductions in plant biomass due to salt water intrusion can have cascading effects on the entire ecosystem (McKee, 1989). These ecological effects could have many important and unforeseeable impacts on humans. As salts are becoming more commonplace in a host of products such as toothpaste, road salts, not to mention all of the food waste humans collectively make, we can expect environmental salinity levels to continue to rise due to pollution and water runoff. By understanding these processes, we may better prepare for and mitigate these effects.
References
Acosta-Motos, J., Ortuño, M., Bernal-Vicente, A., Diaz-Vivancos, P., Sanchez-Blanco, M., & Hernandez, J. Plant responses to salt stress: adaptive mechanisms. Agronomy, 7(1), 18, (2017).
Cheeseman, J. M. Mechanisms of Salinity Tolerance in Plants. Journal of Plant Physiology, 87(3), 547-550 (1988).
Kaushal, S. S., Groffman, P. M., Likens, G. E., Belt, K. T., Stack, W. P., & Kelly, G. T. Increased salinization of freshwater in the northeastern United States. Proceedings of the National Academy of Sciences, 102(38), 13517-13520, (2005).
McKee, K. L., & Mendelssohn, I. A. Response of a freshwater marsh plant community to increased salinity and increased water level. Journal of Aquatic Botany, 34(4), 301-316, (2003).
Negrão, S., Schmöckel, S. M., & Tester, M. Evaluating physiological responses of plants to salinity stress. Annals of Botany, 119(1), 1-11, (2017).
Nishida, K., Khan, N. M., & Shiozawa, S. Effects of salt accumulation on the leaf water potential and transpiration rate of pot-grown wheat with a controlled saline groundwater table. Soil Science and Plant Nutrition, 55(3), 375-384, (2009).
Silva, E. N., Ribeiro, R. V., Ferreira-Silva, S.L., Viegas, R. A., & Silveira, J.G. Comparative effects of salinity and water stress on photosynthesis, water relations and growth of Jatropha curcas plants. Journal of Arid Environments, 74(10), 1130-1137, (2010).
Strotmann, R., Harteneck, C., Nunnenmacher, K., Schultz, G., & Plant, T. D. OTRPC4, a nonselective cation channel that confers sensitivity to extracellular osmolarity. Journal of Nature and Cell Biology, 2(10), 695-702, (2000).
Thien, L. B. Floral Biology of Magnolia. American Journal of Botany, 61(1), 1037-1045, (1974).
Acknowledgments
I would like to acknowledge Aaron Lenihan for his dedicated help throughout the experimental study as well as his thorough, helpful advice the writing process. I would also like to acknowledge my team members.